Gulden Camci-Unal Develops New Biomaterials for Growing Tissue in the Lab
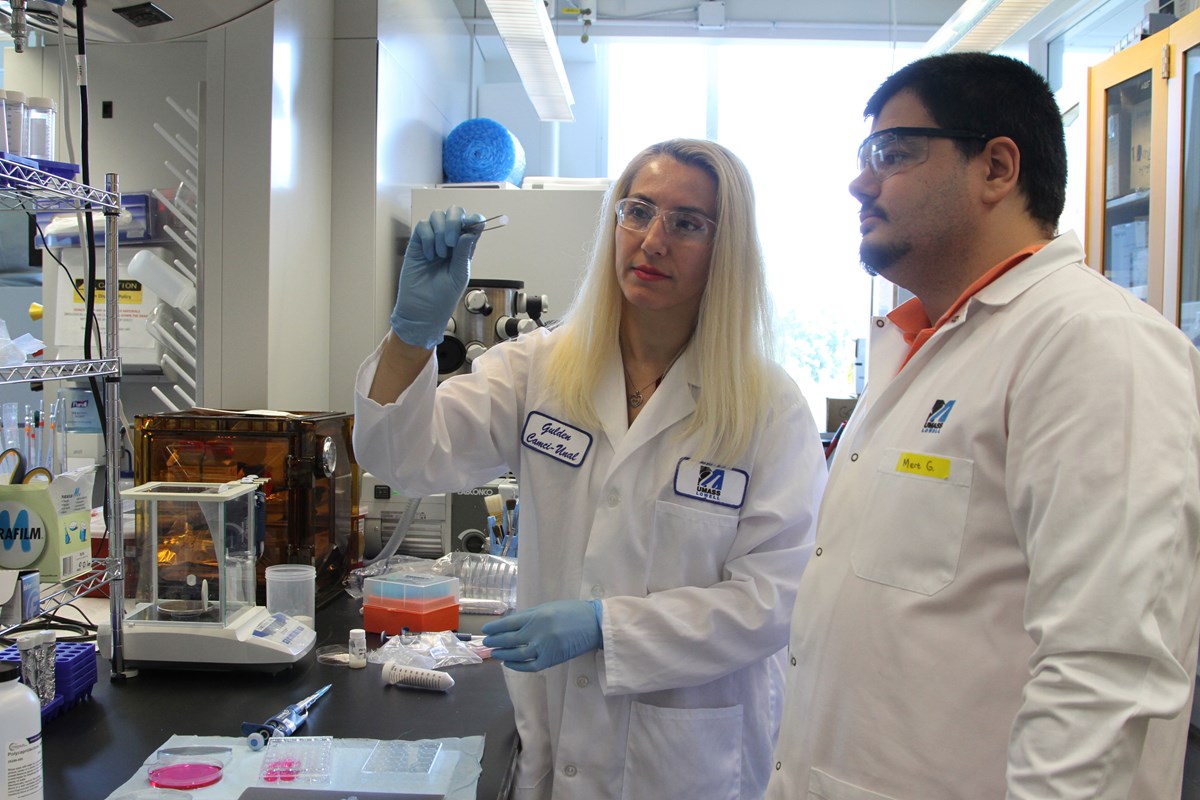
09/01/2024
By Edwin L. Aguirre
In recent years, there have been tremendous advances in tissue engineering, the field of biomaterials research and development that combines living cells with 3D microstructures and biologically active molecules to grow fully functional tissues. Scientists, engineers and physicians are applying tissue engineering technologies such as microfabrication techniques to develop tissue replacement therapies in patients to restore, maintain or improve damaged tissues or whole organs such as bones, the liver or the heart.
Among the researchers at the forefront of this cutting-edge bioengineering technology is Prof. Gulden Camci-Unal of the Department of Chemical Engineering.
Last year, Camci-Unal and her collaborators started developing bioartificial pancreas-like engineered tissues that could someday help to improve the quality of life for people with diabetes. Their study is funded by a three-year grant worth nearly $242,000 from the National Science Foundation.
This year, Camci-Unal and her students are using eggshell particles and 3D printing to grow bone tissue in the lab. The technique can be used for bone grafts and regenerating cartilage, teeth and tendons.
Engineering Biocompatible Materials for Tissue Implantation
Diabetes is a chronic disease in which the pancreas does not produce enough insulin or the body does not respond properly to the insulin that it does produce. As a result, blood sugar, or glucose, always stays high, which can lead to serious, lifelong health complications.
According to the American Diabetes Association, more than 11% of the U.S. population—over 37 million people—has diabetes, and 1.4 million more are diagnosed with the disease each year. The annual economic cost of diabetes in the country runs into hundreds of billions of dollars.
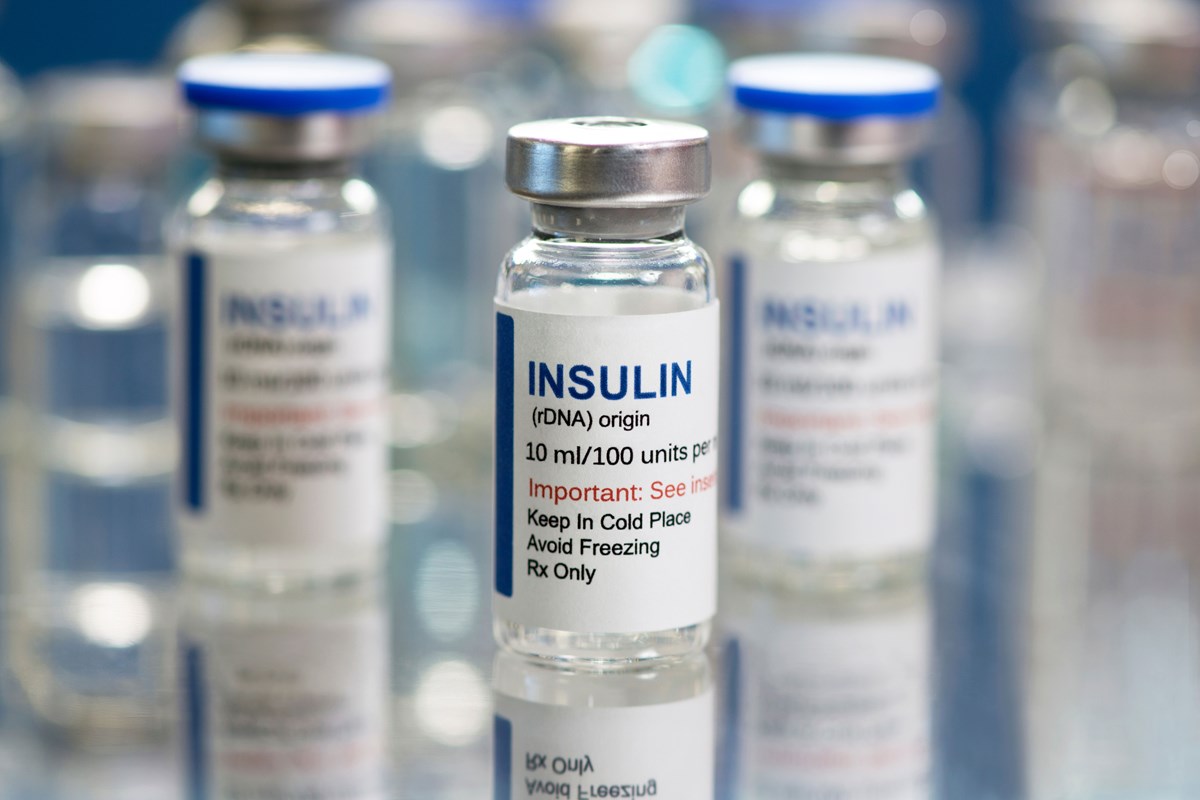
“Individuals with Type 1 diabetes and more than 30% of those with Type 2 diabetes depend on daily injections of insulin,” says Camci-Unal, who is the principal investigator on the grant for UMass Lowell. “However, maintaining optimal blood glucose levels remains a challenge and does not prevent severe long-term complications.”
Camci-Unal says the project will use innovative biomaterials as well as cell and tissue engineering to design and create microscopic 3D structures or scaffolds that will support the growth of insulin-producing pancreatic cells, called beta cells. The ultimate goal is to implant these cell-laden scaffolds into the body to help the patient manage glucose levels more effectively than daily insulin injections.
According to the researchers, the implantable engineered pancreatic tissues that they are developing require a significant number of beta cells as well as a supporting network of blood vessels for the tissues to survive and produce insulin. One way to reduce the required number of beta cells without decreasing insulin output from the tissues is to stimulate the engineered cells’ function by exposing them to light.
“My lab will be taking the lead in developing the appropriate synthetic biomaterials and facilitating the formation of blood vessels inside the scaffolds,” notes Camci-Unal, who is the Robert and Gail Ward Endowed Professor in Biomedical Materials Development.

The researchers will use hydrolyzed collagen-based hydrogels to design and construct the scaffolds and encapsulate the engineered beta cells to protect them from the body’s immune system. The team will also incorporate oxygen-generating compounds as well as cells from the lining of blood vessels (called endothelial cells) into the scaffolds to help promote the formation of the vascular network.
“In addition to diabetes, our scaffolds have the potential for applications in engineering various other tissues such as muscle, liver and neural tissues,” Camci-Unal says.
Collaborating on the project is Prof. Emmanuel (Manolis) Tzanakakis of Tufts University. Camci-Unal’s Ph.D. student, Gokalp Kurtoglu, is assisting with the lab research at UML.
Repurposing Eggshells for Personalized Medicine
Since 2016, Camci-Unal has been conducting tissue engineering research in her lab using finely crushed eggshells to create the scaffolds where bone cells can grow and proliferate. The resulting bone tissue can then be implanted in patients to repair, replace or regenerate diseased or damaged bones and cartilage. These bone defects, which can stem from congenital anomalies, trauma, fractures and surgical removal of tumors, have long posed significant challenges to a person’s quality of life and self-esteem.
According to Camci-Unal, eggshells are exceptionally well-suited for bone tissue engineering applications due to their unique chemical composition, which is primarily calcium carbonate.
“Each year, millions of tons of eggshells are routinely discarded worldwide. Since they are considered as waste, their potential as unconventional biomaterial is largely overlooked in the medical field,” she says. “Our goal is to repurpose this eggshell waste for personalized medicine.”
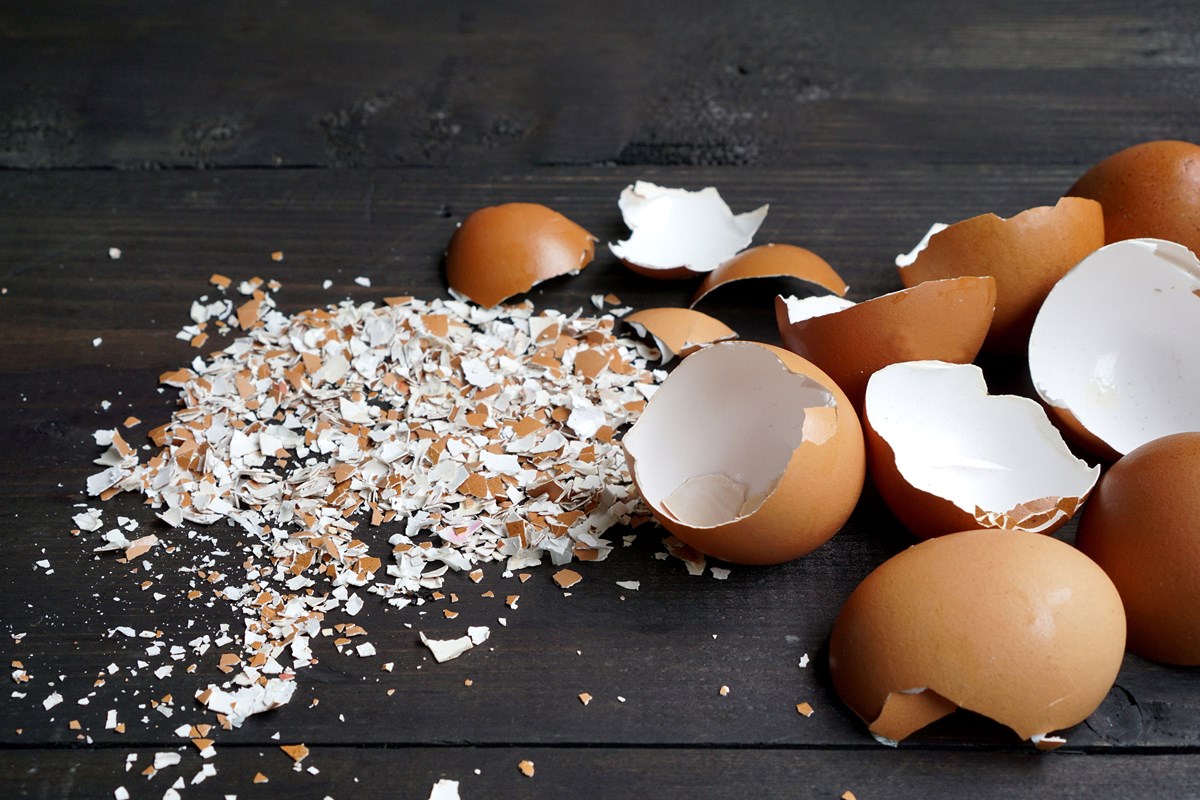
Most recently, Camci-Unal has been using a biocompatible and biodegradable synthetic polymer to strengthen the eggshell-based scaffolds, as well as employing 3D printing technique to create precisely shaped structures for implanting.
“Lab-grown bone grafts reinforced with eggshell microparticles not only support bone growth and healing, but they can also lessen the risk of complications, thereby reducing hospital stay and hastening recovery,” she says.
Aside from bone tissue engineering, other potential uses for eggshell-reinforced scaffold composites include dental restorations as well as skull, face and jaw repairs, bone-to-cartilage reconnections, cartilage regeneration and musculoskeletal applications.
Harnessing the Power of 3D Printing Technology
Initially, Camci-Unal used gelatin-based hydrogels reinforced with eggshell microparticles to create the bone tissue scaffolds for implanting.
This time, she is mixing the powdered eggshells with a hard thermoplastic polymer called polycaprolactone and then feeding the eggshell-polymer “ink” into a 3D printer to build the scaffolds. The resulting structures have much higher mechanical strength and load-bearing capacity than those made with hydrogels.
“The precision of 3D printing technology will enable us to design customized and intricate structures tailored to the individual patient’s needs,” she notes.
Assisting Camci-Unal in the lab research are biomedical engineering and biotechnology Ph.D. student Mert Gezek, chemical engineering postdoctoral researcher Mine Altunbek and undergraduate student Maria Eduarda Torres Gouveia. The team’s preliminary findings on 3D-printed scaffolds were published in June in the peer-reviewed journal ACS Applied Materials & Interfaces.